First published February 2020
Find out why it’s important to correctly assess assimilation lighting and how to make effective use of it, including a look at some of the recent technological developments and advances in plant science.
Quick links:
- The development of LED technology
- Why use assimilation lighting?
- How to use assimilation lighting
- Technological developments
- Plant research advances
- Financial implications
- Light emission legislation
- How is light measured?
- What does the future hold?
The development of LED technology
Light Emitting Diode (LED) lighting has been developed for horticulture since the late 1980s, yet it remains on the periphery of mainstream sources for glasshouse lighting. Conventional assimilation (supplementary) lights, such as high-pressure sodium (HPS) lamps, still tend to be the go-to option for many growers for various reasons.
We consider the developments in LED technology in recent years and whether or not LEDs are an economically viable alternative to conventional light sources.
Why use assimilation lighting?
What are the main reasons for using supplementary lighting in horticulture and how to plants ‘see’ light compared to the human eye?
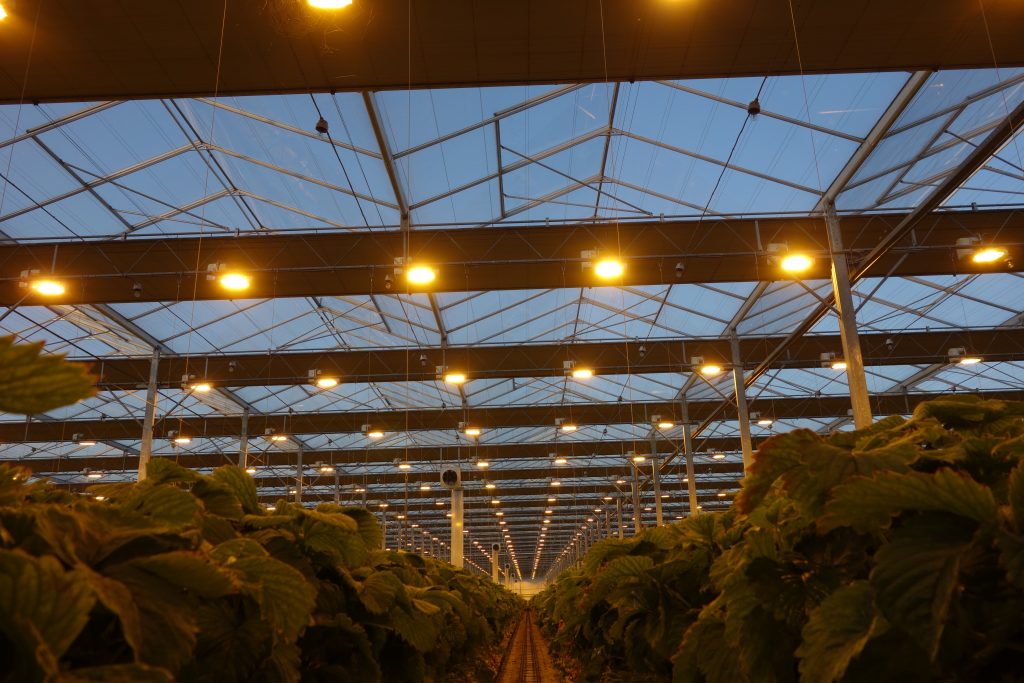
Light is generally considered to be one of the most important factors for effective growing in glasshouses. It is a source of information for plants, with intensity, direction, duration and spectral composition all playing a role. Sunlight contains everything a plant needs for photosynthesis, and has the added bonus of being free. However, the sun doesn’t always shine, and, since growers like to have a degree of regularity and control over their plants and growing conditions, this has necessitated the use of additional artificial sources known as assimilation or supplementary lighting.
The primary reasons to use assimilation lighting are to increase crop production, in terms of yield and by extending the season and improving crop quality. The main aim is not necessarily to replicate sunlight per se but to provide the wavelengths that stimulate specific plant photoreceptors. The spectrum of light seen by the human eye, i.e. visible light in the range of 380-700nm (Figure 1), is different from that ‘seen’ by plants (Figure 2). While the eye perceives a spectrum of colours from violet to red, plants can detect beyond this range. In addition to certain wavelengths of visible light, some plants may need UV light (280-400nm) and/or far-red (700-800nm) light.
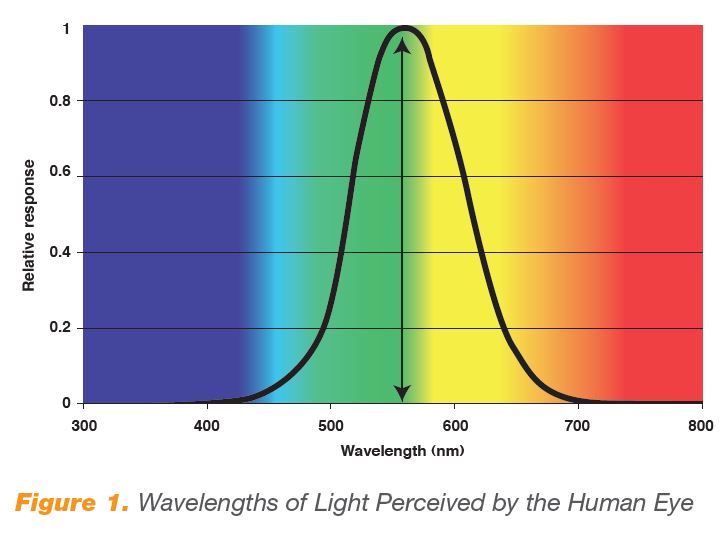
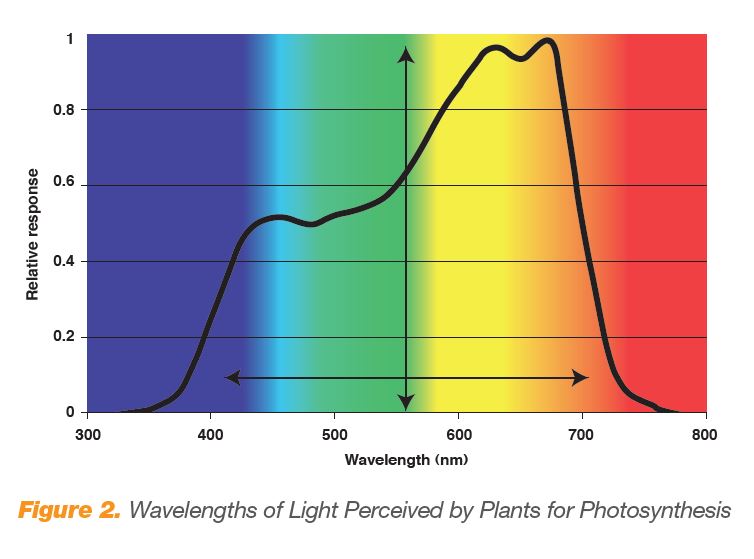
How to use assimilation lighting
How do LEDs compare with high-pressure sodium lamps and what are the practical benefits of LEDs for horticulture?
High-pressure sodium (HPS)
With the benefits of increased crop yield and quality, the use of artificial lighting has become commonplace. Traditionally, high-pressure sodium (HPS) lamps have been used. These are a relatively cheap option and can produce much of the light required by plants. Due to a low degree of efficiency (defined as electrical energy input to light output), HPS lamps generate a large amount of heat. In itself, this isn’t necessarily a bad thing, given that many crops have a high heat requirement. Indeed, this additional ‘free’ heat radiated by the lights is often a welcome byproduct, and can mean fewer operating hours for the designated heating system. It should be noted, though, that this is not a cost-effective method of heating a glasshouse.
How do LEDs differ?
n contrast, LEDs operate at much lower temperatures. Although significant amounts of heat can still be produced, it is dissipated through convection above the lights, rather than through radiation onto the crop. The up-side of less heat generation is a much higher efficiency of the lighting system, meaning lower operating costs. Glasshouses lit with LED lighting systems are expected to require an increased heating requirement of approximately 10% in comparison with HPS lit systems.
Other LED lighting benefits
The light spectrum emitted by LEDs can be adjusted to target the absorption characteristics of the plants, which varies from crop to crop. Typically, a combination of blue, red and far-red light will be required.
In addition, more versatile control strategies can be achieved with LEDs, including rapidly switching on and off, dimmable output and outputting specific wavelengths. Spectral composition can be changed through cropping so, for example, plants may need more blue light early in the season to control morphology and then, later, a higher percentage of red to aid flowering. Furthermore, low levels of radiated heat mean LEDs can be positioned closer to the canopy than HPS lamps, meaning light can reach further into the crop canopy than could be achieved with conventional toplighting. Being able to tailor the light output to the crop requirements is a big advantage in terms of efficiency; high-efficiency results in lower operating costs, with LEDs offering savings of around 30% compared to conventional assimilation lighting systems.
Lifespan of LEDs
Another factor to consider when choosing a lighting system is its lifespan. LEDs have proved themselves to be a reliable technology, but their output will decrease over time. It’s estimated that output drops to around 90% after 25,000 hours (manufacturer’s data) and 85% after 50,000 hours. In contrast, HPS lamps have a survival rate of around 50% after 20,000 hours. Failure of a lamp means replacement and additional cost. Such factors should be considered when determining the payback of an assimilation lighting system.
The financial payback of LED lights is still a lengthy one, however, due to the high capital costs of the system. This can be a stumbling block for growers, despite the fact that LEDs are often reported to increase crop growth and yield compared to HPS lamps.
Technological developments
How the efficiency of LEDs has increased and other technological advances such as electronic ballasts, over-powering of lamps, internal reflector lamps, and new reflective materials.
Efficiency of LEDs
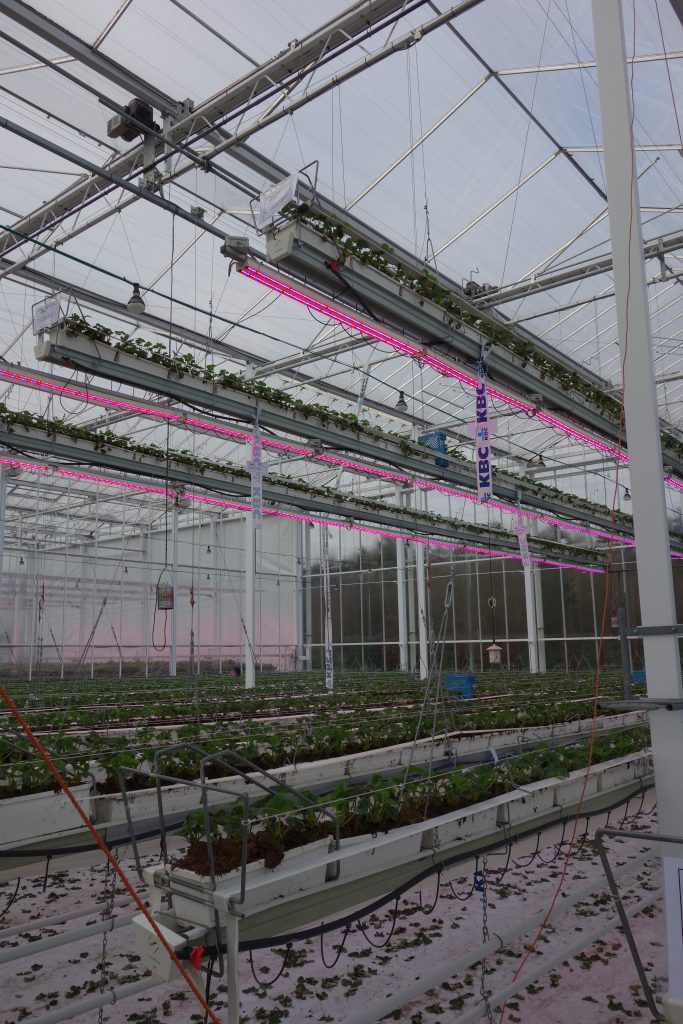
The research into LEDs over the last couple of decades has seen great advancements in the technology. The most notable development is in the efficiency of LEDs, which have a typical output of 2.70 μmol J-1, compared to HPS at 1.85 μmol J-1. The latest generation of interlights can now achieve an output of 3.0 μmol J-1 (Table 1). LEDs allow interlighting to be placed close to the crop at locations where light from standard toplights would not reach.

Other technological advances
AHDB Technical Guide Supplementary Lighting suggests several recent technological advances of interest:
- Electronic ballasts are already commercially available for lower power HID lamps, and larger ones are now being developed. They offer a variety of benefits including less variation in lamp output due to mains voltage effects, lower losses, improved starting, better power factor and lower harmonics.
- Over-powering of lamps: Some luminaire manufacturers are operating 400W lamps at up to 550W and 600W lamps at up to 750W. The claimed advantage of this development is that the output is shifted toward the red (longer wavelength) end of the spectrum and PAR efficiency is improved. In some cases, total PAR output can be increased by over 35%. However, running lamps at well over their rated power will significantly reduce their life. The precise effects on lamp life are currently unknown.
- Internal reflector lamps: A lamp with a built-in reflector has recently been launched. The claims being made by the manufacturer include:
- An improvement in the effective downwards light output ratio (DLOR) of the lamp and luminaire combination.
- Easier maintenance. No independent data is currently available to substantiate these claims, however.
- New reflective materials: Manufacturers are developing polymers that have the potential to improve the performance of reflectors and reduce the effects of ageing. There are currently difficulties in practical applications including problems with the manufacture of reflectors using deepdrawing methods. Also, the polymers cannot withstand the high operating temperatures associated with commercial supplementary lighting equipment.
LED efficacy
AHDB Horticultural Fellowship project CP 085 investigated the implications of ongoing increases in LED efficacy. The maximum theoretical LED efficacy for all colours of light was calculated. Red LEDs had the potential to produce almost 50% more photons than blue LEDs, because red photons contain less energy than blue photons. Currently, red and blue LED technologies to achieve efficacies that are about half the theoretical maximum, demonstrating the potential for considerable future improvements in LED efficacy.
Plant research advances
What can we learn from three specific research studies looking at light and its effect on crops?
Light recipes for specific plants
Manufacturers are not only working on improving efficiency of fixed spectral output LEDs, but also on the development of controllable/programmable units and research specific products. This means there can be high research costs for suppliers, which have not helped to lower the price of more standard units for the market.
There has been considerable research into creating specific plant ‘light recipes’ for many common crops in the academia, commerce and on nurseries. A large body of knowledge is being established, but there is plenty more to learn in developing optimum programmes.
Nelson & Bugbee (2014)
Nelson & Bugbee (2014) compared the photosynthetic (400–700nm) photon efficiency and photon distribution pattern of two double-ended HPS fixtures, five mogul-base HPS fixtures, 10 LED fixtures, three ceramic metal halide fixtures, and two fluorescent fixtures. The two most efficient LED and the two most efficient double-ended HPS fixtures had nearly identical efficiencies at 1.66 to 1.70 micromoles per joule.
These four fixtures represent a dramatic improvement over the 1.02 micromoles per joule efficiency of the mogul-base HPS fixtures that are in common use. The most efficient HPS and LED fixtures had equal efficiencies, but the initial capital cost per photon delivered from LED fixtures was five to ten times higher than HPS fixtures.
The high capital cost means that the five-year cost of LED fixtures was more than double that of HPS fixtures. They advised that if widely-spaced benches were a necessary part of a production system, LED fixtures can provide precision delivery of photons and data indicate that they can be a more cost-effective option for supplemental greenhouse lighting.
AHDB project CP 139 (2015)
AHDB project CP 139 (2015) compared seven different LED, plasma and HPS lighting systems measuring electrical efficiency and spectral output. The lights tested were a selection donated by manufacturers, not a comprehensive side-by-side trial, and each had different spectral outputs.
The results showed that three LEDs were more efficient at producing PAR than the benchmark HPS; two LEDs and plasma were less efficient. The spectral properties are also important, and the plasma and LEDs tested had a close match to the solar spectrum.
Spectral output diagrams can be found in Appendix 1 of AHDB Technical Guide Lighting in Practice.
AHDB project CP 125 (2016)
Research that was undertaken in the AHDB project CP 125 (2016) has investigated the impact of lamp spectral outputs on different crops.
Research highlights included: maximum energy efficiencies were achieved at ~200 μmol m-2 s-1, plant growth rate was greatest under mixtures containing ~10% blue light, and maximum growth regulation was achieved under light mixtures containing 30-60% blue light on the crops tested.
Further information can be found in the report and the paper on photobiology in protected horticulture.
Financial implications
How do the costs and efficiency of different types of lighting unit affect economic return?
The unit costs and performance figures from a leading LED supplier, comparing advancements between 2013 and 2018, are summarised in Table 2.
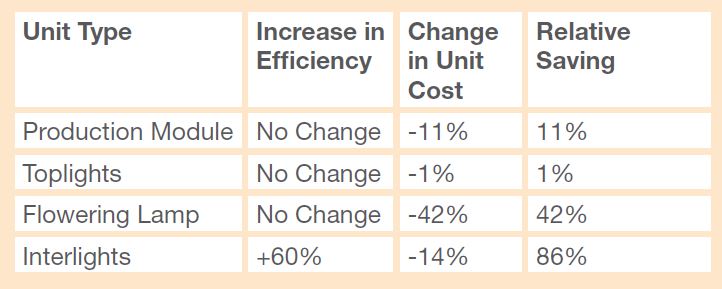
With the latest generation of interlighting, additional savings could be achievable. Although the cost per micromole output remains the same, the efficiency improvements mean less energy is required, hence reduced operating costs. Increased output from the latest units could also mean fewer lights are required in total, which helps to lower installation costs.
Table 3 contains some indicative costs for the different unit types, as well as the Photon Flux output. These example figures can be used to estimate total cost for an LED setup, based on individual requirements of light output.
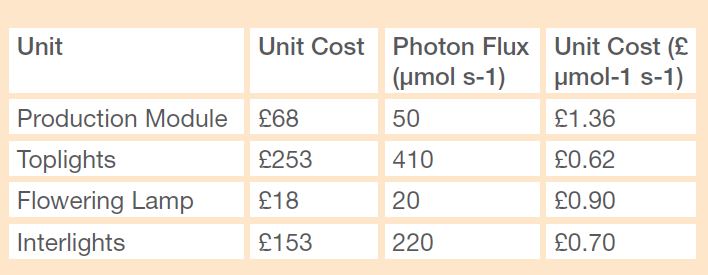
For comparison, for larger projects (> 2 Ha), a fully installed LED toplight setup can cost three to four times as much as equivalent HPS fixtures; approximate costs are £120/m2 for LEDs and £33/m2 for HPS.
AHDB project PO 010 investigated the economics of lighting options in 2012. The calculators have since been updated as part of the CP 085 Horticultural Fellowship and are now available on the GrowSave website to allow growers to compare various offerings in the market place. There are also practical guidelines to help growers select the best equipment in the Supplementary lighting technical guide.
Light emission legislation
Any future regulation of light emissions could play a role in working out which technology to choose.
While not yet a concern to growers in the UK, the regulation of light emissions could play a role in determining which technology to choose. Growers in The Netherlands, where regulations are enforced, use screens to limit light emissions. If using HPS, this can result in the build-up of heat in the glasshouse to undesirable levels, resulting in crop quality issues. Such problems can potentially be avoided if using LEDs.
There are currently no European standards for horticultural lighting, adding to difficulties in comparing offerings from different manufacturers. It is hoped this will be addressed and simplify the product choices.
How is light measured?
An explanation of the various light measures.
Table 4 gives an explanation of the various light measures.
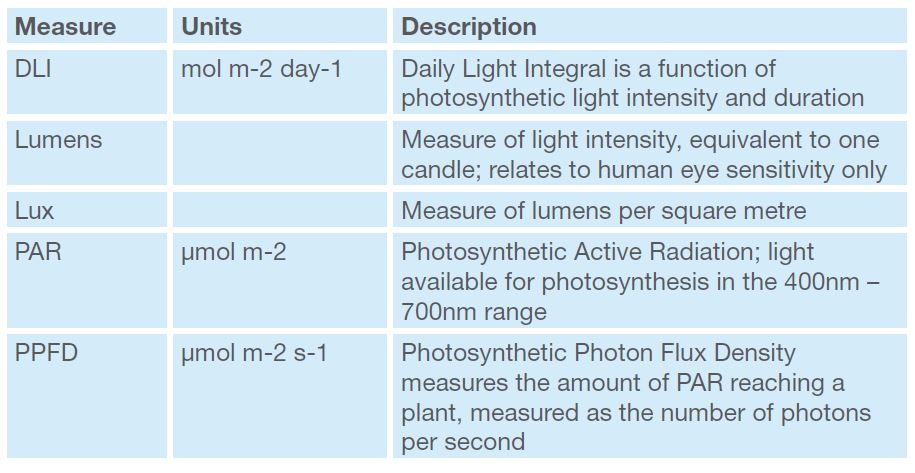
What does the future hold?
As plant science develops and costs associated with LEDs fall, they are likely to gain in popularity and economic viability.
Technology has moved on considerably in recent years. HPS lamps are still the industry standard, but LEDs continue to prove themselves as a viable alternative and are growing in popularity.
The primary benefit of LEDs is their low operating cost, due to being very energy efficient. There is also evidence that LEDs can improve crop quality and yield, but this can be difficult to quantify in financial terms. Compared to HPS lamps, LEDs radiate considerably less heat; while this allows the units to be placed closer to the crop, it can also mean an increase in heat demand from the primary heat source.
Largely due to the financial implications of installing LEDs, the technology has not yet taken off in the way many might have expected. Continued reductions in unit costs will lead to a greater uptake. Furthermore, as the science of plant light recipes are better understood and more research is carried out, it could result in more quantifiable gains in yield and crop quality, compared to conventional lighting.
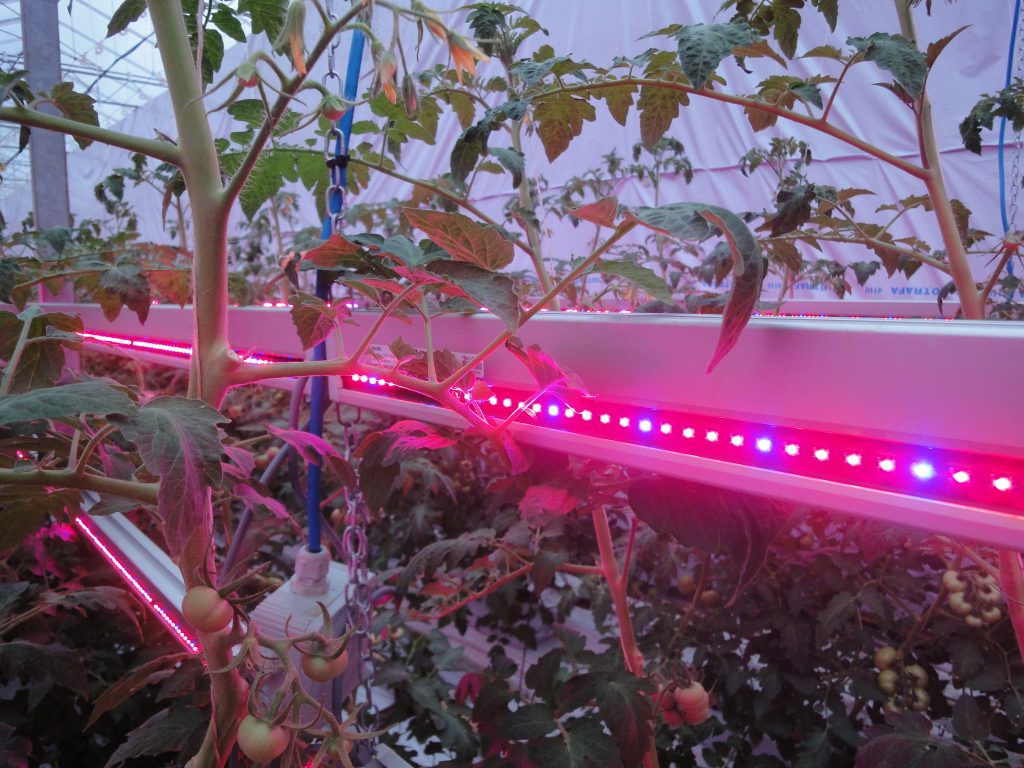